Cell Chemistries
How Cells Work
Galvanic Action
In simple terms, batteries can be considered as electron pumps. The
internal chemical reaction within the battery between the electrolyte and the
negative metal electrode produces a build up of free electrons, each with a negative charge, at the battery's
negative (-) terminal - the anode. The chemical reaction between the electrolyte and the
positive (+) electrode inside the battery produces an excess of positive (+) ions
(atoms that are missing electrons, thus with a net positive charge) at
the positive (+) terminal - the cathode of the battery. The electrical
(pump) pressure or potential difference between the + and - terminals
is called voltage or electromotive force (EMF).
Different
metals have different affinities for electrons. When two dissimilar
metals (or metal compounds) are put in contact or connected through a
conducting medium there is a tendency for electrons to pass from the
metal with the smaller affinity for electrons, which becomes positively
charged, to the metal with the greater affinity which becomes
negatively charged. A potential difference between the metals will
therefore build up until it just balances the tendency of the electron
transfer between the metals. At this point the "equilibrium potential"
is that which balances the difference between the propensity of the two
metals to gain or lose electrons.
A
battery or galvanic cell stores energy in chemical form in its active
materials and can this convert this to electrical energy on demand,
typically by means of an electrochemical oxidation-reduction (redox)
reaction. (Note the generic name "redox" seems to have been
appropriated by a recent flow battery design employing two vanadium
redox couples).
Each galvanic or energy cell consists of at least three and sometimes four components
-
The anode
or negative electrode (the reducing or fuel electrode) which gives up
electrons to the external circuit and is oxidised during the
elecrochemical (discharge) reaction. It is generally a metal or an
alloy but hydrogen is also used. The anodic process is the oxidation of
the metal to form metal ions.
( LEO Lose Electrons - Oxidation)
- The cathode
or positive electrode (the oxidising electrode) which accepts electrons
from the external circuit and is reduced during the electrochemical
(discharge) reaction. It is usually an metallic oxide or a sulfide but
oxygen is also used. The cathodic process is the reduction of the oxide
to leave the metal.
(GER Gain Electrons - Reduction). Remember the mnemonic of the lion growling.
- The electrolyte
(the ionic conductor) which provides the medium for transfer of charge
as ions inside the cell between the anode and cathode. The electrolyte
is typically a solvent containing dissolved chemicals providing ionic
conductivity. It should be a non-conductor of electrons to avoid self
discharge of the cell.
- The separator which electrically isolates the positive and negative electrodes.
The Discharge Process
When
the battery is fully charged there is a surplus of electrons on the
anode giving it a negative charge and a deficit on the cathode giving
it a positive charge resulting in a potential difference across the
cell.
When the circuit is completed
the surplus electrons flow in the external circuit from the negatively
charged anode which loses all its charge to the positively charged
cathode which accepts it, neutralising its positive charge. This action
reduces the potential difference across the cell to zero. The circuit
is completed or balanced by the flow of positive ions in the
electrolyte from the anode to the cathode.
Since
the electrons are negatively charged the electrical current they
represent flows in the opposite direction, from the cathode (positive
terminal) to the anode (negative terminal).
Two Electrolyte Systems
The principles of the Galvanic cell can be demonstrated by the workings of the Daniell cell, a two electrolyte system.
THE CATHODE |
DANIELL CELL |
THE ANODE |
The positive pole of the battery |
|
The negative pole of the battery |
|
Zinc loses electrons more readily than copper |
|
|
Accepts electrons from the external circuit |
Supplies electrons to the external circuit |
|
|
Copper metal deposits on the cathode |
Zinc goes into aqueous solution |
|
|
The site of Reduction |
The site of Oxidation |
|
|
The half-cell with the highest electrode potential |
The half-cell with the lowest electrode potential |
GER |
LEO |
Two electrolyte primary cell systems have been around since 1836 when the Daniell cell was invented to overcome the problems of polarisation. This arrangement illustrates that there are effectively two half cells at which the chemical actions take place. Each electrode is immersed in a different electrolyte with which it reacts. The electrode potential,
either positive or negative, is the voltage developed by the single
electrode. The electrolytes are separated from each other by a salt bridge or porous membrane
which is neutral and takes no part in the reaction. By the process of
osmosis, it allows the sulphate ions to pass but blocks the metalic
ions.
This two electrolyte scheme allows more degrees of freedom or control over the chemical process.
Although
more complex these cells enabled longer life cells to be constructed by
optimising the electrolyte/electrode combination separately at each
electrode.
More recently they have been employed as the basis for Flow Batteries in which the electrolytes are pumped through the battery, providing almost unlimited capacity.
Zinc is a very popular anode material and the chemical action above causes it to dissolve in the electrolyte.
The Daniell cell shown can be said to "burn zinc and deposit copper"
Note-
The simple, single electrolyte cell can also be represented by two half
cells. It can be considered a special case of a Daniell cell with the
two electrolytes being the same.
The
model of the cell as two half cells is used by electrochemists and cell
designers to calculate electrode potentials and and characterise the
chemical reactions within the cell. The cell voltage or electromotive force (EMF)
for the external current derived from a cell is the difference in the
standard electrode potentials of the two half cell reactions under
standard conditions. But real voltaic cells will typically differ from
the standard conditions. The Nernst equation
relates the actual voltage of a chemical cell to the standard electrode
potentials taking into account the temperature and the concentrations
of the reactants and products. The EMF of the cell will decrease as the
concentration of the active chemicals diminishes as they are used up
until one of the chemicals is completely exhausted.
The theoretical energy available from the cell can be calculated using Gibbs free energy equation for the initial and final equilibrium states.
Fortunately
such intimate knowledge of cell chemistry and thermodynamics is not
usually required by the battery applications engineer.
Primary cells
In
primary cells this electrochemical reaction is not reversible. During
discharging the chemical compounds are permanently changed and
electrical energy is released until the original compounds are
completely exhausted. Thus the cells can be used only once.
Secondary cells
In
secondary cells this elecrochemical reaction is reversible and the
original chemical compounds can be reconstituted by the application of
an electrical potential between the electrodes injecting energy into
the cell. Such cells can be discharged and recharged many times.
The Charging Process
The
charger strips electrons from the cathode leaving it with a net
positive charge and forces them onto the anode giving it a negative
charge. The energy pumped into the cell transforms the active chemicals
back to their original state.
Choice of Active Chemicals
The
voltage and current generated by a galvanic cell is directly related to
the types of materials used in the electrodes and electrolyte.
The propensity of an individual metal or metal compound to gain or lose
electrons in relation to another material is known as its electrode
potential. Thus the strengths of oxidizing and reducing agents are
indicated by their standard electrode potentials. Compounds with a
positive electrode potential are used for anodes and those with a
negative electrode potential for cathodes. The larger the difference
between the electrode potentials of the anode and cathode, the greater
the EMF of the cell and the greater the amount of energy that can be
produced by the cell.
Electrochemical Series
is a list or table of metallic elements or ions arranged according to
their electrode potentials. The order shows the tendency of one metal
to reduce the ions of any other metal below it in the series.
A sample from the table of standard potentials shows the extremes of the table.
 |
Strengths of Oxidizing and Reducing Agents
|
 |
Cathode (Reduction)
Half-Reaction |
Standard Potential
E ° (volts) |
Li + (aq) + e - -> Li(s) |
-3.04 |
K + (aq) + e - -> K(s) |
-2.92 |
Ca 2+ (aq) + 2e - -> Ca(s) |
-2.76 |
Na + (aq) + e - -> Na(s) |
-2.71 |
Zn 2+ (aq) + 2e - -> Zn(s) |
-0.76 |
Cu 2+ (aq) + 2e - -> Cu(s) |
0.34 |
O 3 (g) + 2H + (aq) + 2e - -> O 2 (g) + H 2 O(l) |
2.07 |
F 2 (g) + 2e - -> 2F - (aq) |
2.87 |
|
The
values for the table entries are reduction potentials, so lithium at
the top of the list has the most negative number, indicating that it is
the strongest reducing agent. The strongest oxidizing agent is fluorine
with the largest positive number for standard electrode potential.
The
table below shows some common chemicals used for battery electrodes
arranged in order of their relative electrode potentials.
 |
Anode Materials |
 |
|
 |
Cathode Materials |
 |
(Negative Terminals) |
(Positive Terminals) |
BEST - Most Negative |
BEST Most Positive |
Lithium |
Ferrate |
Magnesium |
Iron Oxide |
Aluminum |
Cuprous Oxide |
Zinc |
Iodate |
Chromium |
Cupric Oxide |
Iron |
Mercuric Oxide |
Nickel |
Cobaltic Oxide |
Tin |
Manganese Dioxide |
Lead |
Lead Dioxide |
Hydrogen |
Silver Oxide |
Copper |
Oxygen |
Silver |
Nickel Oxyhydroxide |
Palladium |
Nickel Dioxide |
Mercury |
Silver Peroxide |
Platinum |
Permanganate |
Gold |
Bromate |
WORST Least Negative |
WORST Least Positive |
Cells
using aqueous (containing water) electrolytes are limited in voltgage
to less than 2 Volts because the oxygen and hydrogen in water
dissociate in the presence of voltages above this voltage. Lithium
batteries (see below) which use non-aqueous electrolytes do not have
this problem and are available in voltages between 2.7 and 3.7 Volts.
However the use of non-aqueous electrolytes results in those cells
having a relatively high internal impedance.
Alternative chemical reactions
More recently new cell chemistries have been developed using alternative chemical reactions to the traditional redox scheme.
Metal Hydride Cells
Metal
hydride cell chemistry depends on the ability of some metals to absorb
large quantities of hydrogen. These metallic alloys, termed hydrides,
can provide a storage sink of hydrogen that can reversibly react in
battery cell chemistry. Such metals or alloys are used for the negative
electrodes.The positive electrode is Nickel hydroxide as in NiCad
batteries. The electrolyte, which is also a hydrogen absorbent aqueous
solution such as potassium hydroxide, takes no part in the reaction but
serves to transport the hydrogen between the electrodes.
Lithium Ion Cells
Rather
than the traditional redox galvanic action, Lithium ion secondary cell
chemistry depends on an "intercalation" mechanism . This involves the
insertion of lithium ions into the crystalline lattice of the host
electrode without changing its crystal structure. These electrodes have
two key properties
- Open crystal structures which allow the the insertion or extraction of lithium ions
- The ability to accept compensating electrons at the same time
Such electrodes are called intercalation hosts.
In
a typical Lithium cell, the anode or negative electrode is based on
Carbon and the cathode or positive electrode is made from Lithium
Cobalt Dioxide or Lthium Manganese Dioxide. (Other chemistries are also
possible)
Since Lithium reacts
violently with water, the electrolyte is composed of non aqueous
organic Lithium salts and acts purely as a conducting medium and does
not take part in the chemical action, and since no water is involved in
the chemical action, the evolution of hydrogen and oxygen gases, as in
many other batteries, is also eliminated.
During
discharge lithium ions are dissociated from the anode and migrate
across the electrolyte and are inserted into the crystal structure of
the host compound. At the same time the compensating electrons travel
in the external circuit and are accepted by the host to balance the
reaction.
The process is completely
reversible. Thus the lithium ions pass back and forth between the
electrodes during charging and discharging. This has given rise to the
names "Rocking chair", "Swing" or "Shuttlecock" cells for the lithium
ion batteries.
Variations
on the Lithium technology are also used in primary cells which were
originally developed for space and military applications. These include
Lithium-thionyl chloride and Lithium-sulphur dioxide chemistries which
use reactive electrolytes and liquid cathodes to obtain higher energy
and power densities.
Alternative chemistries - Special flavours
Designing
a better battery is not simply a matter of choosing a pair of elements
with a larger difference in electrode potentials, there are many other
factors which come into play. These may be: availability and cost of
the raw materials, stability or safety of the chemical mix,
manufacturability of the components, reversibility of the
electrochemical reaction, conductivity of the components, operating
temperature range and quite possibly the desire to circumvent some
other manufacturer's patent. All of these considerations lead to the
use a limited range of basic chemistries but with a wider variety of
proprietary material formulations.
Over
the years a wide range of cell chemistries and additives has been
developed to optimise cell performance for different applications.
Alternative
active compounds may be substituted to increase energy densities (See
below), increase the current capacity, reduce internal impedance,
reduce the self discharge, increase the terminal voltage, improve the
coulombic efficiency or reduce costs.
Additional
compounds may be incorporated to modify the behaviour of the active
compounds to increase cycle life, to prevent corrosion or leakage, to
control polarisation or to increase safety. These could include
catalysts which may be used to promote or accelerate desired chemical
actions such as recombination of the active chemicals in sealed cells.
They could also include inhibitors which may be added to slow down or
prevent unwanted physical or chemical actions such as the formation of
dendrites.
Added
to the range of available cell chemistries are the different cell
capacities and physical constructions of the cells, the battery
applications engineer thus has a wide variety of options from which to
choose.
Energy Density
The
energy density is a measure of the amount of energy per unit weight or
per unit volume which can be stored in a battery. Thus for a given
weight or volume a higher energy density cell chemistry will store more
energy or alternatively for a given storage capacity a higher energy
density cell will be smaller and lighter. The chart below shows some
typical examples.
Relative Energy Density of Some Common Secondary Cell Chemistries
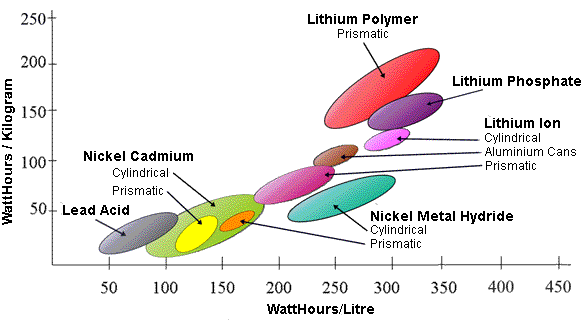
In
general higher energy densities are obtained by using more reactive
chemicals. The downside is that more reactive chemicals tend to be
unstable and may require special safety precautions.
The energy density is also dependent on the quality of the active
materials used in cell construction with impurities limiting the cell
capacities which can be achieved. This is why cells from different
manufacturers with similar cell chemistries and similar construction
may have a different energy content and discharge performance.
Note
that there is often a difference between cylindrical and prismatic
cells. This is because the quoted energy density does not usually refer
to the chemicals alone but to the whole cell, taking into account the
cell casing materials and the connections. Energy density is thus
influenced or limited by the practicalities of cell construcion.
Supply of the Basic Chemical Elements
Worried about the availability of exotic chemicals and the effect future demand may have on prices?
The chart below shows the relative abundance of chemical elements in the earth's crust.
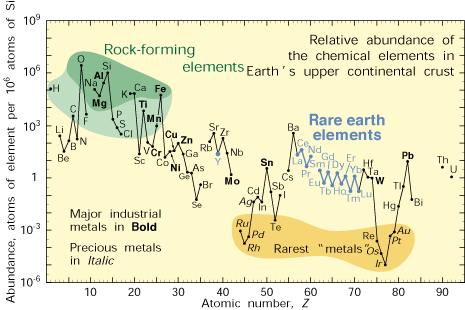
Source - U.S. Geological Survey Fact Sheet 087-02
Note - From the chart above Lithium is between 20 and 100 times more
abundant than Lead and Nickel. The reason it is less common is that
Lithium, being much more reactive than either metal, is not usually
found in its free state, but is combined with other elements. By
contrast Lead being less reactive is more often found in its free state
and is easier to extract and purify. The heavy metals Cadmium and
Mercury whose use is now deprecated because of their toxicity are 1000
times less common than Lithium.
Toxicity of Lithium
In
case you wondered whether there were any toxic effects associated with
Lithium, it is claimed that Lithium on the contrary has theraputic
benefits. The soft drink "7Up" started life in 1929, two months before
the Wall Street Crash, with the catchy name "Bib Label Lithiated
Lemon-Lime Soda". "7Up" contained Lithium Citrate until 1950 when it
was reformulated, some say because of Lithium's association with mental
illness. Since the 1940s, Lithium in the form of Lithium Carbonate has
been used successfully in the treatment of mental disorder particularly
manic depression. As with most chemicals however, small doses may be
safe or theraputic, but too much can be fatal.
Make your own battery at home or at school
See Homebrew Batteries for instructions on how to make a battery using simple materials available at home.
Practical Cell Chemistries
Some
of the most common cell chemistries are described and the applications
for which they are suitable if you follow the links below:-
Primary Cells
Secondary Cells
Unusual Batteries
Cell chemistry Comparison Chart
Alternative Energy Generation and Storage Methods
|